Amongst the many extraordinary properties of graphene, its giant diamagnetic response at zero doping, predicted in the 1950s, had to this day not been measured directly. By controlling the doping of a nearly defect-free graphene sheet and using ultra-sensitive giant magnetoresistance sensors, physicists have for the first time demonstrated this magnetic singularity.
Electrons in a magnetic field follow circular orbits, generating a magnetic moment that opposes the magnetic field. This effect gives rise, in its quantum version, to the well-known Landau diamagnetism of conducting materials. This orbital magnetism has long been known to depend on the specific crystalline and electronic structure of the material considered. It has more recently been noted that the electronic wavefunction acquires a phase in the course of the electron’s motion. This phase, of geometrical origin, called the Berry phase, reflects the crystal symmetries, and affects the sign of the magnetic response.
In the case of graphene, the electronic structure possesses a remarkable feature, the Dirac point, where two cone-shaped energy bands meet, where the electrons behave as if their mass were zero. As early as 1956, a diamagnetic divergent orbital response was predicted when the chemical potential coincides with the Dirac point energy, i.e. when the lower band is completely filled with electrons and the upper band completely empty (figure). This prediction is surprising, since the electron density is minimal precisely at this Dirac point. The response can be understood considering the p Berry phase of graphene, which causes a negative orbital response, thus opposing the magnetic field and hence diamagnetic. Moreover, this phase also enters in the quantization of the Landau energy levels, leading to a level at the Dirac point energy, thus creating a non-zero density of states at this point. The first experimental evidence of this magnetic singularity has just been provided by a collaboration between physicists from the Laboratoire de Physique des Solides in Orsay (LPS, CNRS/Université Paris-Saclay), using an ultrasensitive measurement of the magnetization of a practically defect-free crystalline flake of graphene a few microns in size, and controlling the electron density around the Dirac point with an electrostatic field. This study is published in the journal Science. See also the News and Views (in French) published on the CNRS website.
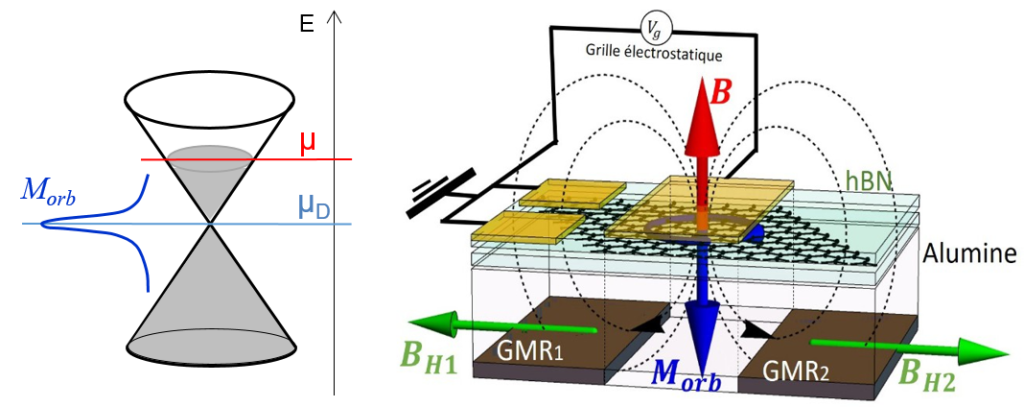
Figure. (Left) Schematic of the two cone-shaped bands at the Dirac point: the electronic energy E is shown as a function of the vector characterizing momentum. The shaded areas indicate the electron filling, which defines the chemical potential (μ). When μ = μD (μD energy at the Dirac point), the lower band is completely filled and the upper band completely empty. The blue curve represents the diamagnetic peak of the orbital magnetic moment (Morb) measured when μ = μD. (Right) Schematic of the experimental setup that detected the diamagnetic anomaly in graphene at the Dirac point. The graphene sheet is protected by two layers of boron nitride (hBN) and placed on an alumina substrate. The arrows represent the exciting magnetic field (red), the orbital response of the electrons (blue) and the fields detected by the giant magnetoresistance sensors (green). The potential Vg is applied to a grid to control the chemical potential.
The measurement device consists of a pair of giant magnetoresistance (GMR) sensors fabricated at the SPEC laboratory (CEA/CNRS) which feature an extremely high sensitivity to the magnetic field generated by the orbital current loops induced in the graphene, all the while being insensitive to the magnetic field perpendicular to the graphene plane that generates those loops (figure). An electrostatic gate electrode, placed above the sample, controlled graphene’s chemical potential. The high susceptibility of graphene‘s magnetic response to doping was exploited by modulating the gate voltage periodically with time, and detecting the synchronous GMR signal. This achieved an optimal discrimination of the sought-after magnetic signal from the spurious noises of the experiment: the accuracy obtained is better than one millionth of the earth’s magnetic field. In addition, indeed, as the gate voltage was changed to bring the chemical potential in the range of the Dirac point, a diamagnetic peak was detected at the Dirac point, in agreement with theory. With increasing magnetic field, the peak width increases and periodic oscillations appear on both sides of the Dirac point, corresponding to de Haas-van Alphen oscillations, which are the signatures of other Landau energy levels.
These results open a whole new field of investigation of orbital currents in graphene and in two-dimensional topological materials. They offer a new tool to explore correlated electronic states generated by the combined effect of Coulomb interactions, strain and moiré potentials.
Reference
Detection of graphene’s divergent orbital diamagnetism at the Dirac point
J. Vallejo, N.J. Wu, C. Fermon, M. Pannetier-Lecoeur, T. Wakamura, K. Watanabe, T. Tanigushi, T.Pellegrin, A. Bernard, S. Daddinounou, V. Bouchiat, S. Guéron, M. Ferrier, G. Montambaux, H. Bouchiat
Science, 2021, 21, 10178-10185
doi: 10.1126/science.abf9396
Open Access repository: arXiv
News & Views from CNRS
Contact
Hélène Bouchiat