The coincidences in time between the energy loss of an electron traversing a material and a subsequent light emission allow the measurement of the relative quantum efficiency of the material. An experimental setup was built at Laboratoire de Physique des Solides, which detects electrons and photons one-by-one with nanosecond and nanometer precision.
Understanding the energy transfer, diffusion and re-emission processes in materials is fundamental to optimizing materials for different applications. Typically, optical spectroscopies are used to probe those processes. Those techniques have a limited spatial resolution and detection energy range (from the infrared to the ultraviolet). By contrast, electron spectroscopies using fast electron with picometer wavelengths, offer a spatial resolution below one nanometer with a typical energy detection range extending from the far infrared (~20 meV) to the hard X-ray (> keV). However, electron spectroscopy techniques have some limitations. An important one is the broadband nature of electron scattering. In short, the energy transferred in each scattering process cannot be known a priori, but only the average scattering spectrum for an ensemble of electron scattering events.
In a study published in Science Advances, researchers from LPS in collaboration with a team from Tsukuba (Japan) circumvented this limit by detecting the energy lost by each electron interacting with a sample. The originality of this work is that they could, along with the energy lost, detect the electron scattering arrival time at the detector with sub 10 ns precision. With this information, they measured the probability to detect the emission of a photon as a function of the energy lost. This probability allowed detecting unknown excitation channels leading to light emission when fast electrons interact with semiconductors. Indeed, it is a wide spread hypothesis in electron spectroscopy, supported by experimental evidence but without an ultimate proof, that most of the light emitted from semiconductors excited by electron stem from the decay of bulk plasmons. With these measurements, the team confirmed that core-electron excitations can also lead to light emission and that unexpectedly near band edge excitations are very efficient towards photon generation (Figure). They could demonstrate this by studying with nanometer resolution hexagonal boron nitride thin flakes and core-shell Au/SiO2 spheres. All this work was made possible by the development of a specific detector for the EELS (Y. Auad, et al., Ultramicroscopy, 239, 113539 (2021)) in a way that the arrival of a photon on a photomultiplier tube could be time-tagged on the same universal clock as the arrival of electrons on the detector.
This work is a first step towards the understanding of energy transfer process in solids excited by fast electrons. It opens the way to a quantitative measurement of the quantum efficiency at the nanometer, a key parameter in all light emitting materials/technologies. A direct extension of this work involves the measurement of lifetimes from the electron-photon coincidence decay curves.
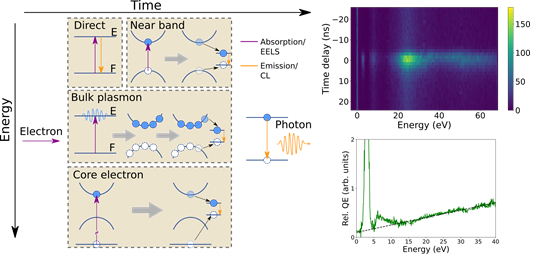
Left: schematic of the process leading to cathodoluminescence (CL, photon emission in orange) starting from an electron energy loss event (EELS, in purple). Upper right: 2D histogram of electron-photon coincidence events as a function of time and energy. Lower right: relative quantum efficiency of h-BN as a function of energy. The extra quantum efficiency at around 6 eV, associated to near-band edge transitions, was not known to be an efficient pathway for light emission under fast electron excitation.
Reference
Cathodoluminescence excitation spectroscopy: Nanoscale imaging of excitation pathways
N. Varkentina, Y. Auad, S. Y. Woo, A. Zobelli, L. Bocher, J. D. Blazit, X. Li, M. Tencé, K. Watanabe, T. Taniguchi, O. Stéphan, M. Kociak, L. H. G. Tizei
Science Advances, 2022, 8, eabq4947
doi: 10.1126/sciadv.abq4947
Contact
Luiz Tizei